In 1985, scientists vaporized graphite using a laser and looked closely at the residue. What they found was hollow cage like molecules made of carbon with 12 pentagonal and 20 hexagonal faces – much like a soccer ball. These molecules were named fullerenes, and was clear proof that diamond and graphite were not the only stable forms of carbon. A discovery so significant that the scientists were awarded the Nobel prize in 1996. Today, science has advanced enough to allow placing metals inside hollow fullerenes and boy, does that make things more interesting!
Why encage metals?
Metals are extremely unstable at the nano scale and below. Trapping them in a fullerene cage makes them stable. This lets scientists design experiments to understand how nano sized metals interact among themselves, as well as with carbon, which forms the cage. Over time, such studies can lead to new compounds with unprecedented properties. Thus, encaged metals are ideal platforms to explore nanomaterial chemistry.
Fullerenes with encaged metals
Fullerenes form at high temperatures. It is possible to physically insert some atoms and molecules like helium and water into fully formed fullerenes. However, metal insertion happens right when the fullerenes form, not after the fact.
What makes encaging metals in fullerenes interesting is the transfer of charge from metals to the outer carbon cage. Strong interaction between the inner metal and outer carbon cage makes these fullerenes very stable. It also leads to some unique and fascinating phenomena, such as fusing. Electron density on the outer cage fluctuates with the position of metal within. When metals are positioned just right, carbon-carbon bonds form to fuse two fullerene cages.
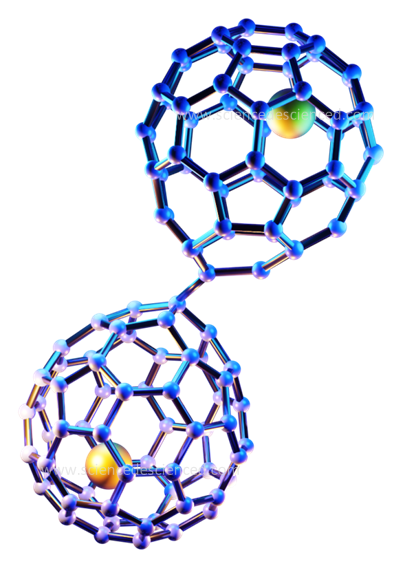
Single metal vs. metal cluster
The most common type of metallofullerene has one metal species. However, even clusters of metals can form the core of metallofullerenes. Clusters of various shapes and sizes have been observed so far. The size and symmetry of the outer cage are what determines the size and shape of a cluster. It may resemble a folded butterfly wing, a zigzag pattern or even lie flat on the cage floor depending on the cage structure! The metallic units within clusters bond among themselves, but the type of bond changes for different fullerene cages. The curved cage also facilitates strong interactions between metals even if they are far apart.
When it comes to shape and symmetry, metallofullerenes are not nearly as perfect as empty fullerenes. That’s a small price to pay though considering the remarkable possibilities metals bring to these structures. Here are some examples:
- Transfer of electrons from metal to the cage to make the fullerene magnetic
- Tailored reactions at specific sites on the cage
- Metal clusters of different sizes give different products on the cage
- Changing the metals inside fullerenes make them more reactive
- Cage reactions control how metals move
When different molecules are attached onto the cage, they alter the geometry of the cage. This affects how the metals (or metal clusters) within bond and move. Some reactions that occur on the cage ring can displace, stop, or change motion of metal clusters within. It also determines whether metals bond or not. Thus controlling cage reactions can let us fine tune how metals in clusters move and bond with other metals.
Final thoughts
Metals encaged in fullerenes are fascinating materials we don’t yet fully understand. However, the field is rapidly evolving and it is only a matter of time before we see more breakthroughs. A clearer understanding of how these structures form will help encage transition metals in fullerenes. Those fullerenes will be even better candidates for efficient catalysis and solid state energy storage solutions!